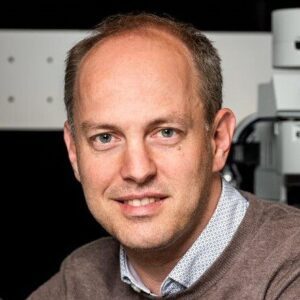
Fundamental process behind memory now captured live
18 September 2023
18 September 2023
Researchers from the Netherlands Institute for Neuroscience have, for the first time, witnessed nerve plasticity in the axon in motion.
Our nerve cells communicate through rapid transmission of electrical signals known as action potentials. All action potentials in the brain start in one unique small area of the cell: the axon initial segment (AIS). This is the very first part of the axon, the long, thin extension of a nerve cell that transmits signals or impulses from one nerve cell to another. It acts as a control center where it is decided when an action potential is initiated before traveling further along the axon.
Previously, researchers made the surprising observation that plasticity also occurs at the AIS. Plasticity refers to the brain’s ability to create new connections and structures in order to scale the amount of electrical activity, which is crucial for learning and memory. AIS plasticity occurs during changes in brain network activity. The segment’s length can become shorter with excessive activity or longer with low activity. But how does this structure change, and how quickly does it happen? Amélie Fréal and Nora Jamann in the lab of Maarten Kole have, for the first time, observed in real-time how this adaptability functions within the axon and identified the molecular mechanisms behind this process.
Key players in this process are ion gates located at the segment, also known as sodium channels. The team developed new tools to study these sodium channels and their supporting proteins. They discovered that the number of sodium channels in the cell’s segment can change rapidly, within an hour. This rapid change is mediated by a process called endocytosis, in which the sodium channels are taken up into vesicles within the cell. Nora Jamann explains, “You can think of this adaptability as a kind of amplifier that allows you to fine-tune the input. The longer the AIS, the less current you need. You can enhance the cell’s output. If this isn’t properly adjusted, learning can be compromised.”
Figure: When there is low activity in the network, the number of sodium channels in the plasma membrane increases to amplify the output. With high activity in the network, the sodium channels are internalised into the cell through ‘endocytosis’.
“When you learn, the activity in the neural network constantly fluctuates. Neurons need to operate with extreme variations in levels of activity—both too low and too high activity can be harmful for memory formation.’ Her previous experiments in mice illustrate this adaptability: a mouse with clipped whiskers receives reduced sensory input. As a result, the number of sodium channels in the AIS increases to maintain balance. The opposite also occurs: if there is too much input, such as when the mouse is placed in a new environment with high activity, the AIS becomes slightly shorter and has fewer sodium channels. But how this works and how fast this plasticity occurs have always been fundamental questions for neuroscientists.
Amélie Fréal says, “To address this question, we faced a significant challenge: how can we capture plasticity live? If you wonder how the AIS adapts, you want to actually see it moving. This was not previously possible in the field. In this research, we used two new tools: first, a special mouse model with the AIS labeled with a fluorescent protein, allowing us to observe the mechanism and record the temporal changes in brain slices. Second, we used molecular tools that made sodium channels visible in cell cultures. This made it possible to track the sodium channels live for the first time.”
Jamann continues, “The plasticity we observe in the AIS closely resembles what we know about synaptic plasticity. This is the most well-known form of plasticity and relates to the ability of the connection (the synapse) between two nerve cells to change in strength. Synaptic plasticity is directly linked to learning and memory. But the same mechanism also occurs in the AIS.” Amélie Fréal adds, “If you want to be a bit provocative, you can even say that a lot of change occurs in the synapse, but only the relevant information is forwarded to the next nerve cell. This decision is made in the AIS, so changes in this region are genuinely important for the cell’s function.”
Fréal concludes, “This study brings together different areas of expertise, which I strongly support in research. Maarten Kole’s lab has all the equipment to record and see the activity, and I came with novel tools to image the nanoscale structures. Together, we have been able to establish that when activity changes, structure changes as well. This observation will help us see plasticity in a broader perspective. When looking at plasticity in general, we now need to consider these changes in the AIS. Through this collaboration, we have been able to combine our strengths to advance knowledge. That’s what I’m most proud of.”
Source: Science Advances
The Friends Foundation facilitates groundbreaking brain research. You can help us with that.
Support our work