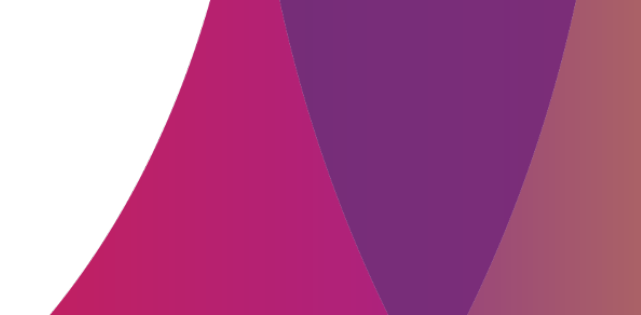
Attention and working memory
Emergence of complex behaviour can only occur if an organism has the ability to perceive, learn and store experiences from its environment. In our lab we are interested in learning the mechanism by which our brain accomplishes complex phenomenon such as attention and working memory. In a stimulus rich environment attention provides a powerful mechanism for selective information processing, while working memory which is special form of short term memory allows retention of critical and task relevant information. Our specific aim is to get a better insight into the interaction between different early visual areas such as V1, V4 and higher visual areas such as frontal eye fields (FEF) during visual attention and working memory tasks. To understand these complex interactions we record neuronal activity from single cell and multiple cells in awake behaving monkeys and mice. We also use techniques such as optogenetics, micro-iontophoresis (drug injection) and pressure injections to manipulate neuronal activity by targeting specific neurotransmitter receptors for acetylcholine, glutamate, dopamine etc. This gives us a deeper understanding into the specific role played by the Cholinergic, Glutamatergic, Dopaminergic etc. neurotransmitter systems during attention and working memory processes.
Decision making and action selection
One of the most fundamental functions of any nervous system is to transform sensory input into motor behavior. In the visual domain, inputs are naturally processed to generate saccadic movements towards the center of objects, which requires a perceptual processing step to isolate objects from the background that has to be read out by the motor system to execute the saccade towards the appropriate position.
Our lab has developed an expertise in several tasks with macaque monkeys that involve saccade towards target objects and has shed light on the neuronal mechanism of the sensory processing in early visual areas. In particular we understand better the neuronal basis of the attentional mechanism underlying the segregation of objects from their background. We are now investigating how this signal is transformed into a motor plan. We are targeting in particular the superior colliculus (SC), which stands out as an excellent candidate for this function. This midbrain structure is organized into layers that receive inputs from V1 and project towards motor structures. The neurons from the superficial layers seem to be mainly responsive to visual inputs, but the deep layer present visuomotor neurons that are active not only in response to visual stimuli in their receptive field but also to saccades towards it. The deep layers are also connected with higher order cortical areas that represent goals. Thus, the SC has an ideal position in the brain to merge sensory information from bottom-up sources with top-down goal-related signals to select an appropriate motor response. We are using single and laminar electrodes to investigate how attentional and sensory information are represented and transformed into a motor command across the different layers.
Reward processing
A reward is an appetitive stimulus that typically serves as a reinforcer. When presented after a particular behavior, it causes the probability of that behavior’s occurrence to increase. By acting as motivational tool and inducing feelings of pleasure, it promotes learning, decision making and goal-directed behavior.
Classically, reward processing has been attributed to brain regions like Ventral Tegmental Area (VTA), responsible for generating incentive motivation and approach towards rewarding stimuli. A subset of the neurons in this regions mediate dopaminergic neurotransmission. However, neuronal correlates of reward processing are also observed in different brain regions, even in the primary visual cortex (area V1). Do these effects of reward in visual cortex depend on cholinergic neuromodulatory signals from basal forebrain? Recent evidence from our lab (Stanisor, van der Togt, Pennartz & Roelfsema, PNAS, 2013) showed that there is a unified signal for reward and attention in primary visual cortex. To further understand the relation between attention and reward processing we now study the role of neuromodulatory signals during selective attention and reward perception in visual areas of the brain.
To tackle these question, we employ several state of the art techniques, such as electrophysiology (multi-electrode and laminar probe recording), two-photon and wide-field calcium imaging in awake, behaving animals to understand the functional architecture underlying reward driven behavior.
Learning and plasticity
Adaptation to the environment is vital for survival. This can be achieved by natural selection but the process happens throughout generations and is very slow. Another way to adapt is to change reactions to stimuli in the environment to more beneficial ones. This process requires two things: Advanced sensory systems as the stimuli as well as the consequences have to be perceived and a plastic computational apparatus that can relate stimuli to consequences and select among behaviors.
We assume that learning to map sensory stimuli to behavior leads to changes in the responses of many neurons involved in the process. Our hypothesis is that these changes are facilitated by attention, and that the learning processes in the visual system require attention to be paid to a stimulus in order to learn its meaning efficiently. We are interested in understanding which regions of the cortex show plasticity during the learning process and how these regions interact in order to change the behavior. We believe that the interplay of feed-forward and feed-back connectivity in the cortex is required for learning.
We use a combination of wide-field imaging and electrophysiology in awake behaving mice to get an idea about the processes and their changes happening in the whole cortex while rather simple tasks are learned. In order to understand the interactions between attention and learning we use UTAH arrays to record single- and multi-unit activity in areas V1 and V4 of macaque monkeys. Both methods allow gathering data from multiple brain regions simultaneously which is important to establish temporal relationships between the processes happening in different regions of the cortex.
Consciousness
Understanding how consciousness emerges in the brain is one of the major challenges that remain to be addressed in neuroscience. What is the difference between information that reaches awareness and information that does not?
To investigate this we have subjects detect and report a brief stimulus that is presented near the threshold for perception. Although the stimulus remains constant from trial to trial, the subject’s perception of the stimulus changes from trial to trial because the stimulus is very hard to perceive. This way, neural responses corresponding to the subject’s perceptual report cannot be attributed to changes in the physical stimulus but must instead reflect conscious perception. By using this paradigm and recording activity from neurons in higher visual area V4 with receptive fields that overlap with a phosphene that is elicited by very well controlled electrical microstimulation (MS) of neurons in primary visual cortex (V1), we directly compare information transfer along the visual cortical hierarchy between perceived and identical non-perceived phosphenes. Studies like these will shed light on the differences between conscious and non-consciousness processing, thereby giving us more insight into the mechanisms that determine perception and one day hopefully enabling us to explain how consciousness emerges in the brain.
Dual task interference
Most people experience difficulties to perform more than one task at once. Generally, this leads to suboptimal performance; the response to one of the tasks is slowed and/ or more mistakes are made. For example, when two tasks have to be performed with a short delay between them, the reaction time for the second task is delayed (i.e. the psychological refractory period). There are numerous theories on the processes that cause the inability to perform two tasks completely in parallel. Some studies suggest that the sharing of a common resource between two tasks functions as a bottleneck and therefore causes this delay. Others suggest that the selection of two responses cannot happen in parallel. However, it is not clear which neural mechanisms underlies these behavioral outcomes.
We study how the activity in different areas along the visual hierarchy (i.e. V1, V4 and FEF) is affected when a monkey performs two tasks. We use multielectrodearrayelectrode arrays to record single-unit and multi-unit activity to get an insight in the neural basis of dual task interference in these areas. An example of an electrode array is shown on the picture with a penny to indicate the size of the array. Since we record in areas along the visual hierarchy we can study dual task interference at different levels of stimulus processing (i.e sensory activation, visuo-motor transformation, response selection and motor output).
Additional information and articles
Vacancies
At the moment, there are no vacancies within this group
Show all vacanciesContact
"*" indicates required fields
Donate to NiN
"*" indicates required fields
Brain Friends make groundbreaking brain research possible
- You support groundbreaking/ innovative brain research
- You support the Dutch Brain Bank
- Invitation to the Brain Friends Lecture
- Exclusive friend activities
- A look behind the scenes of the Netherlands Institute for Neuroscience
You can easily donate via
You can also transfer your contribution to NL76 INGB 0002 1673 78 in the name of ‘Stichting Vrienden van het Herseninstituut’
Support our work!
The Friends Foundation facilitates groundbreaking brain research. You can help us with that.
Support our work